Seed Structure and Anatomy
The structure, anatomy and morphology of mature seeds: an overview
The structure, anatomy and morphology of mature seeds: model species in seed biology
Endospermic seed structure (Eudicots): Brassicaceae - Arabidopsis thaliana
Endospermic seed structure (Eudicots): Brassicaceae - Lepidium sativum
Endospermic seed structure (Eudicots): Cestroidae subgroup of Solanaceae - tobacco and other Nicotiana-species
Endospermic seed structure (Eudicots): Solanoidae subgroup of Solanaceae - tomato and pepper
Endospermic seed structure (Eudicots): Euphorbiaceae - castor bean - Ricinus communis
Non-endospermic seed structure (Eudicots): Fabaceae - pea and other leguminosae
Perispermic seed structure (Eudicots): Amaranthaceae - sugar beet - Beta vulgaris
Endospermic seed structure (Monocots): Alliaceae - onion
Endospermic seed structure (Monocots): Poaceae (cereal grain, caryopsis) - wheat and other cereals
 |
 |
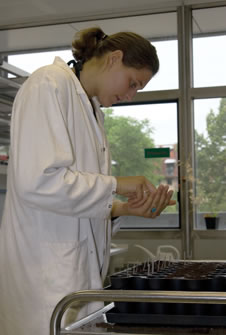
Lab work: Julia is sowing seeds.
Photographer: Johannes Fehrle |
|
 |
|
|
The structure, anatomy and morphology of mature seeds: an overview
More general seed structural features:
- Seeds are the dispersal and propagation units of the Spermatophyta (seed plants): Gymnosperms (conifers and related clades) and Angiosperms (flowering plants). A comparison of these to major groups is presented on the "Seed evolution" webpage. Several seed-related issues differ between gymnosperms (750 species) and angiosperms (250000 species). If not otherwise stated, the information given in this website refers to typical Angiosperm seeds.
- Seeds are mature, fertilized ovules. Ovules are structures of seed plants containing the female gametophyte with the egg cell, all being surrounded by the nucellus and 1-2 integuments. In angiosperms the double fertilization results in formation of the diploid embryo and the triploid endosperm.
- Embryo: Young sporophyt, dipoid (2n), result of fertilization. The mature embryo consists of cotyledons (seed leaves), hypocotyl (stem-like embryonic axis below the cotyledons), radicle (embryonic root).
- Seed and embryo types were defined by Martin (1946). These and the resulting evolutionary trends are found on the "seed evolution" webpage.
- Endosperm: Food storage tissue, triploid (3n), result of double fertilization, 2/3 of the genome is of maternal origin.
- Testa (seed coat): Outer protective layer of the seed, developed from the integuments of the ovule, diploid maternal tissue.
- Fruits are mature, ripened ovaries containing seeds. The pericarp ("fruit coat") is diploid maternal tissue.
- Perisperm: Diploid maternal food storage tissue originates from the nucellus. Only in some species, e.g. Beta vulgaris, Piper nigrum, Coffea arabica, many Caryophyllales.
- Endospermic seeds: The endosperm is present in the mature seed and serves as food storage organ. Testa and endosperm are the two covering layers of the embryo. The amount of endosperm in mature seeds is highly species-dependent and varies from an abundant endosperm layer (Nicotiana tabaccum) to a single layer (Arabidopsis thaliana).
- Non-endospermic seeds: The cotyledons serve as sole food storage organs as in the case of pea (Pisum sativum). During embryo development the cotyledons absorb the food reserves from the endosperm. The endosperm is almost degraded in the mature seed and the embryo is enclosed by the testa.
- Seed types B1, B2, B3, B4, LA, P, UD, FA1, FA2, FA3, FA4 (Martin et al. 1946, revised by Baskin and Baskin 2007): Based on internal morphology of the embryo and endosperm, see 'seed evolution webpage 2'.
More special seed structural features:
- Hilum and funiculus: Funicular scar on seed coat that marks the point at which the seed was attached via the funiculus to the ovary tissue.
- Micropyle: The Micropyle is a canal or hole in the coverings (seed coat) of the nucellus through which the pollen tube usually passes during fertilization. Later, when the seed matures and starts to germinate, the micropyle serves as a minute pore through which water enters. The micropylar seed end has been demonstrated to be the major entry point for water during tobacco seed imbibition and germination. During germination the tobacco testa ruptures at the micropylar end and the radicle protrudes through the micropylar endosperm.
- Chalaza: Non-micropylar end of the seed. The base of an ovule, bearing an embryo sac surrounded by integuments.
- Raphe: Ridge on seed coat formed from adnate funiculus.
- Arillate: General term for an outgrowth from the funiculus, seed coat or chalaza; or a fleshy seed coat.
- Aril: Outgrowth of funiculus, raphe, or integuments; or fleshy integuments or seed coat, a sarcotesta. Arils probably often aid seed dispersal, by drawing attention to the seed after the fruit has dehisced, and by providing food as an attractant reward to the disperser. The aril of the nutmeg produces the spice mace and the seed itself is the nutmeg.
- Strophiole: Outgrowth of the hilum region which restricts water movement into and out of some seeds. In some hard-coated legume seeds, e.g. Melilotus alba and Trigonella arabica, a plug covering a special opening - the strophiolar cleft - must be loosened or removed before water can enter, and then only through this region.
- Operculum: A little seed lid. It refers to a dehiscent cap of a seed or a fruit that opens during germination.
- Carunculate: Seed with an excrescent outgrowth from integuments near the hilum, as in Euphorbia.
- Fibrous: Seed with stringy or cord-like seed coat, as mace in Myristica.
- Funicular: Seed with a persistent elongate funiculus attached to seed coat, as in Magnolia.
- Strophiolate: Seed with elongate aril or strophiole in the hilum region.
- Fruit: Strictly, the ripened ovary of a plant and its contents. More loosely, the term is extended to the ripened ovary and seeds together with any structure with they are combined, e. g. the apple (a pome) in which the fruit (core) is surrounded by flesh derived from the floral receptacle.
- Achene: A small, usually single-seeded, dry indehiscent fruit, e.g. lettuce.
- Caryopsis: A dry, nut-like fruit typical of grasses, e. g. a cereal grain. It is an achene with the ovary wall united with the seed coat.
- Elaiosomes: A specialty in the dispersal through animals is that through ants (myrmecochory). Such seeds or fruits bear attachments, the elaiosomes that contain lures and nutriments. Myrmecochory is common with plants that live at the forest soil like violets (Viola).
- Caruncle: A reduced aril, in the form of a fleshy, often waxy or oily, outgrowth near the hilum of some seeds. Usually it is brightly colored. It acts as an aid to dispersal. Viola seeds have an oily caruncle and are sought and dispersed by ants.
- Mucilage: A layer of polysaccharide slime produced by some seeds upon imbibition. Serves in water uptake during imbibition and germination.
The structure, anatomy and morphology of mature seeds:
model species in seed biology
Family (clade) |
 |
Examples |
 |
Description |
Endospermic seeds  |
Cucurbitaceae
- Core Eudicots
- Rosid clade
|
 |
muskmelon (Cucumis melo ) |
|
In the muskmelon seed the embryo is surrounded by a perisperm/endosperm envelope. Callose (ß-1,3-glucan) deposition in this envelope is responsible for the apoplastic semipermeability of muskmelon seeds. The perisperm/endosperm envelope is weakened prior to the completion of germination. |
Fabaceae
- Core Eudicots
- Rosid clade
|
 |
fenugreek (Trigonella foenum-graecum)
crimson clover (Trifolium incarnatum)
lucerne (Medicago sativa) |
|
Only some legume (Fabaceae) seeds are endospermic, most legume seeds are non-endospermic. |
Euphorbiaceae
- Core Eudicots
- Rosid clade
|
 |
castor bean (Ricinus communis) |
|
Castor bean seeds (Malpighiales) are a classical seed system to study endosperm reserve breakdown. |
Brassicaceae
- Core Eudicots
- Rosid clade
|
 |
garden cress (Lepidium sativum)
mouse-ear cress (Arabidopsis thaliana) |
|
Only some Brassicaceae seeds are endospermic, most Brassicaceae seeds are non-endospermic. Mature seeds of Lepidium have 1-2 cell layers of endosperm, while Arabidopsis has a single endosperm cell layer. These two species exhibit, as tobacco, a two-step germination (distinct testa rupture and endosperm rupture). We found that Lepidium is a promising model system for endosperm weakening Müller et al. 2006). |
Solanaceae
- Core Eudicots
- Asterid clade
|
 |
Cestroideae subgroup:
tobacco (Nicotiana tabacum)
other Nicotiana-species
petunia (Petunia hybrida)
Solanoideae subgroup:
tomato (Lycopersicon esculentum)
pepper (Capsicum annuum)
Datura (Datura ferox)
|
|
Mature seeds of the Solanaceae family usually have an abundant endosperm layer. Well investigated examples are tobacco and tomato, which are model systems in seed biology for the study of endosperm weakening and the regulation of germination by plant hormones and environmental factors. The Solanaceae family can be divided into two large subgroups:
Cestroideae subgroup of Solanaceae (tobacco, petunia):
Straight or slightly bent embryos and prismatic to subglobose seeds, two-step germination (distinct testa rupture and endosperm rupture), typically capsules as fruits.
Solanoideae subgroup of Solanaceae (pepper, tomato):
Curved embryos and flattened, discoid seeds, no visible distinction beween testa rupture and endosperm rupture, often berries as fruits. |
Rubiaceae
- Core Eudicots
- Asterid clade |
 |
Coffee (Coffea arabica) |
|
The coffee embryo is enveloped by an endosperm tissue. The fully differentiated embryo lies inside an embryo cavity. The endosperm is surrounded by endocarp, which resembles a seed coat. Endosperm weakening of coffee is inhibited by abscisic acid (ABA) and promoted by gibberellins (GA). |
Oleaeceae
- Core Eudicots
- Asterid clade |
 |
Syringa species |
|
Low temperature dormancy of Syringa seeds is mainly imposed by the mechanical resistance of the endosperm layer. |
Asteraceae
- Core Eudicots
- Asterid clade |
 |
lettuce (Lactuca sativa) |
|
Lettuce "seeds" are actually fruits and have 2-3 cell layers of endosperm below the pericarp. Lettuce endosperm weakening has been demonstrated and the hormonal regulation of lettuce seed germination is similar to tobacco. |
Apiaceae
- Core Eudicots
- Asterid clade |
 |
celery (Apium graveolens) |
|
Seeds of Apiaceae (Umbelliferae) contain relatively large amounts of living endosperm which completely surrounds a small embryo located at the micropylar end of the seed. Endosperm breakdown has been demonstrated and is promoted by gibberellins. |
Ranunculaceae
- Basal Eudicots |
 |
Trollius species |
|
The seeds of basal angiosperms often have underdeveloped embryos that are embedded in abundant endosperm tissue. Two-step germination process with distinct testa rupture and endosperm rupture. |
Poaceae and
other monocot families
- Basal Angiosperms
- Monocots |
 |
wheat (Triticum aestivum)
barley (Hordeum vulgare)
maize (Zea mays)
rice (Oryza sativa)
wild oat (Avena fatua)
onion (Allium cepa)
|
|
A typical monocot seed with endosperm is onion (Allium cepa), Alliaceae family. In the highly specialized cereal grains/caryopses (wheat, barley, maize) the endosperm can be divided in the starchy endosperm (starch grains, food storage, dead cells, flour) and the aleurone layer (living cell layer surrounding the starchy endosperm). The cereal embryos are highly specialized in their structure. |
Non-endospermic seeds  |
Fabaceae
- Core Eudicots
- Rosid clade |
 |
pea (Pisum sativum)
garden bean (Phaseolus vulgaris)
soybean (Glycine max) |
|
Most species of the legume family (Fabaceae) including pea (Pisum sativum) and diverse beans have non-endospermic seeds. The cotyledons serve as sole food storage organs as in the case of pea (Pisum sativum). During embryo development the cotyledons absorb the food reserves from the endosperm completely. In the mature seed the embryo is enclosed solely by the testa as the only seed covering layer. The regulation by ethylene of pea seed germination and seedling emergence has been studied in detail. |
Brassicaceae
- Core Eudicots
- Rosid clade |
 |
rape (Brassica napus)
wild mustard (Sinapis alba)
wild radish (Raphanus sativus) |
|
Most species of the mustard family (Brassicaceae) including several Brassica-species have non-endospermic seeds. The cotyledons serve as sole food storage organs as described for the non-endospermic Fabaceae seeds. |
Seeds with perisperm  |
Amaranthaceae
(incl. Chenopodiaceae)
- Core Eudicots
- Caryophyllid clade
|
 |
sugar beet (Beta vulgaris)
lambsquarters (Chenopodium album) |
|
Perisperm is diploid maternal food storage tissue that originates from the nucellus. It is present in mature seeds of many Caryophyllales (Centrospermae) including the Amaranthaceae (Beta, Chenopodium) among the eudicots, but also in basal angiosperms like black pepper (Piper nigrum), Piperaceae. |
 |
 |
 |
|
|
|
Endospermic seed structure (Eudicots): Brassicaceae -
Lepidium sativum as model system in seed biology |
In mature seeds of Lepidium sativum (garden cress) the embryo is surrounded by 1-2 cell layers of endosperm. FA2-type seed.
We found that Lepidium seeds exhibit, as tobacco, a two-step germination process with distinct testa rupture and endosperm rupture.
We found that Lepidium seeds provide an excellent model system for the experimental investigation of endosperm weakening.
Lepidium and Arabidopsis are closely related species and exhibit, except for the size, highly similar seed structure Müller et al. (2006). Besides Arabidopsis and Lepidium, most Brassicaceae seeds are non-endospermic. |
|
|
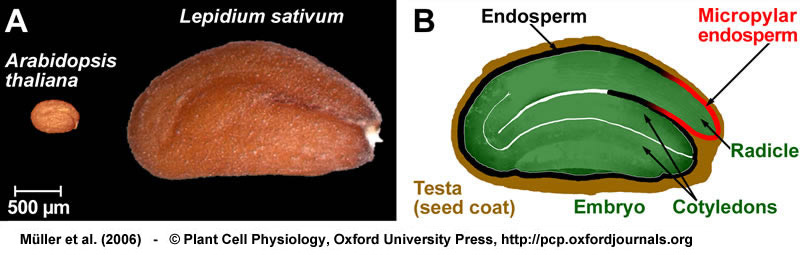
Seeds of Lepidium sativum and Arabidopsis thaliana have similar seed anatomy and similar germination physiology.
(A) The larger size of Lepidium seeds allows methods for which Arabidopsis seeds are too small, e.g. puncture force experiments.
(B) Drawing of a mature Lepidium seed; the embryo is enclosed by the endosperm and the surrounding testa.
From
Müller et al. (2006).
|
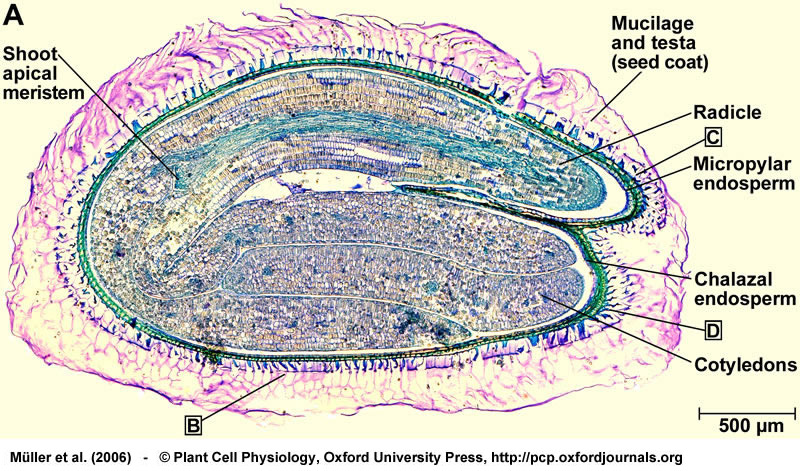
Structure of a mature seed of Lepidium sativum. Bright field microscopy of longitudinal sections of 2-3 h imbibed seeds stained with toluidine blue. (A) Entire seed, showing the mature and fully differentiated embryo, the endosperm, and the testa (seed coat). The boxed letters refer to the positions of the close-up sections. From Müller et al. (2006).
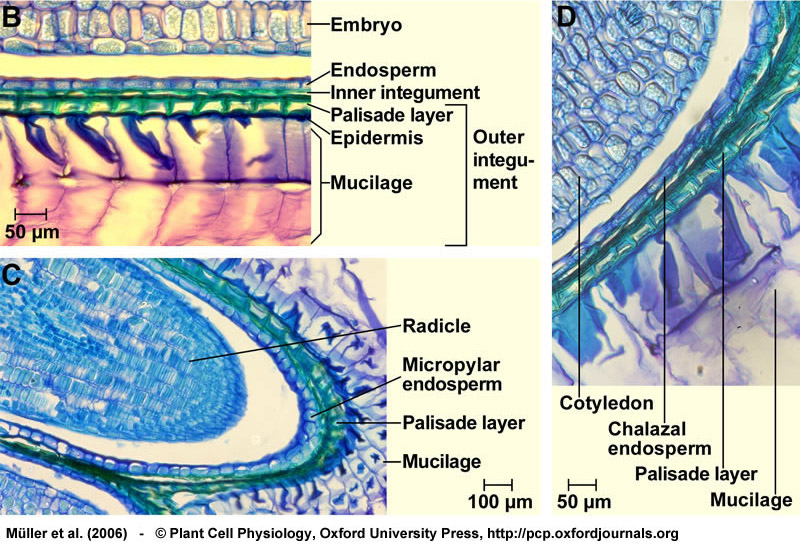
Structure of a mature seed of Lepidium sativum. (B) Structure of the seed covering layers: Endosperm, a single cell layer; and testa (seed coat), composed of inner and outer integument. Note that the mucilage is generated from the outer testa upon imbibition. (C) Structure of the micropylar cap enclosing the radicle tip. The micropylar endosperm has 1-2 cell layers. (D) Structure of the chalazal seed region. From Müller et al. (2006).
|
|
Endospermic seed structure (Eudicots): Brassicaceae -
Arabidopsis thaliana as model system in seed biology |
In mature seeds of Arabidopsis thaliana the embryo is surrounded by a single cell layer of endosperm. FA2-type seed.
Arabidopsis seeds exhibit, as tobacco and Lepidium, a two-step germination process with distinct testa rupture and endosperm rupture, as first described by the group of Hiroyuki Nonogaki (Liu et al., Plant J 41:936-944, 2005).
We found that, as shown for tobacco and Lepidium, ABA inhibits endosperm rupture of Arabidopsis, but does not affect testa rupture (Müller et al., 2006).
The testa is the most important covering layer of Arabidopsis seeds and confers coat dormancy. This has been demonstrated by the Arabidopsis testa mutants by the group of Maarten Koornneef (Debeaujon et al., Plant Physiol 122:404-413 and 415-424, 2000).
Although Arabidopsis does not have a massive endosperm layer, the single endosperm cell layer could be involved in regulating endosperm rupture. Only some Brassicaceae seeds are endospermic (Arabidopsis, Lepidium), most Brassicaceae seeds are non-endospermic.
|
|
|
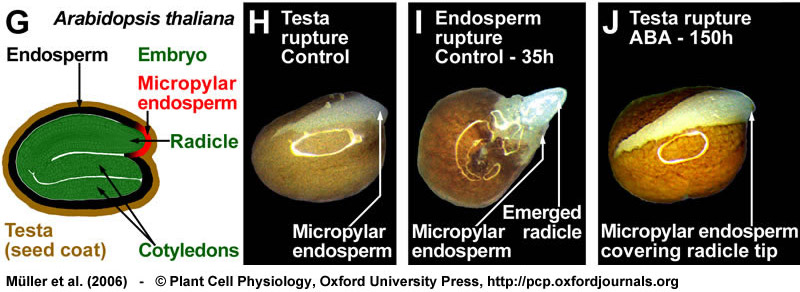
(G) Drawing of a mature Arabidopsis seed; the seed anatomy that is very similar to that of Lepidium. (H-J) Arabidopsis seeds also germinate with testa rupture (H) preceding endosperm rupture (I). Also during the two-step germination process of Arabidopsis, ABA specifically inhibits endosperm rupture (J). Seeds were incubated in continuous light without (control) or with 10 µM ABA added to the medium. From Müller et al., (2006).
A comprehensive table of Arabidopsis hormone mutants summarizes the altered phenotypes regarding germination and dormancy.
|
|
 |
|
|
Endospermic seed structure (Eudicots): Cestroidae subgroup of Solanaceae -
tobacco and other Nicotiana-species as model systems in seed biology |
Nicotiana seeds are the type members of the Cestroideae subgroup of Solanaceae. LA-type seed.
In mature tobacco (N. tabacum) seeds the embryo is surrounded by three to five layers of rather thick-walled endosperm cells in the mature seed. The periphery of the endosperm is pressed against the thin testa (seed coat), which consists of an outer layer of cutinized and lignified dead cells and a living inner parenchyma layer. The maternal origin of theis living cell layer interposed between the endosperm and the dead outer testa is suggested by gene promoter studies and by genetic ablation. The micropylar endosperm surrounds the radicle tip and is the place of radicle protrusion during germination. The embryos are straight or slightly bent and the seeds are prismatic to subglobose (size < 1 mm). For further details with regard to the tobacco seed morphology see the references in the review by Leubner-Metzger (2003).
The publication of Avery (1933) provides excellent drawings of the tobacco seed and seedling structure.
The seed structure of other Nicotiana species and of Petunia species is similar to tobacco. N. attenuata, N. sylvestris and N. plumbaginifolia are other well investigated Nicotiana-species. Testa rupture and endosperm rupture are distinct and temporally separated processes during the seed germination of Nicotiana- and Petunia-species (two-step germination process). For tobacco this two-step germination process has first been observed by Avery (1933), has been re-discovered by Arcila and Mohapatra (1983), and is a general feature of Cestroideae-type seeds (Leubner-Metzger 2003, Petruzzelli et al. 2003). Testa rupture and endosperm rupture are distinct and separate events in many seed species and are an important experimental advantage to pin-point the target sites for hormones ond genes in the germination process.
Both, the testa and the endosperm layer confer coat-imposed dormancy, which can be released by light, gibberellin or cold treatment of imbibed seeds, or by seed after-ripening during dry storage at room temperature.
|
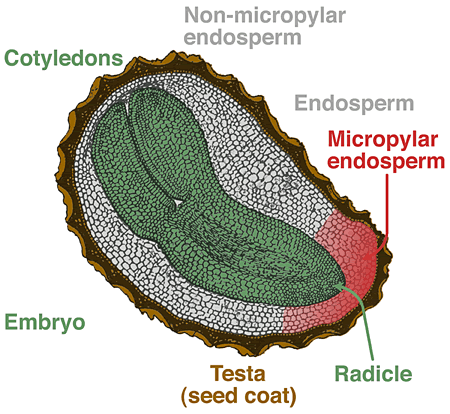 |
Drawing showing a mature seed of Nicotiana rustica (Color image based on a drawing by Ioan Grintescu, In: Gicquet P and Hitier H, La Production du Tabac, J.-B. Baillière et fils, Paris 1961, p. 51). Color drawing published in Finch-Savage and Leubner-Metzger (2006). |
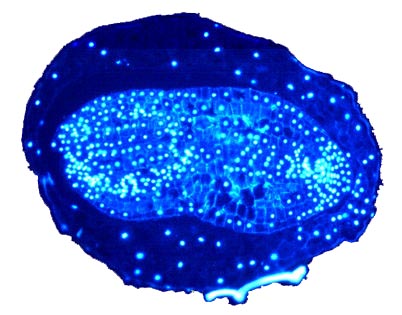
|
Micropscopic picture of a sectioned dry mature Nicotiana tabacum seed: nuclei in the DAPI stain. The swap image "upon mouseover" shows the corresponding brightfield picture. Note that the testa has been removed except for the labeled testa remnant. © 2003 G. Leubner |
Publication and tobacco seed drawings by Avery (1933):
The two drawings below are a redesign by me of Fig. 3K,L,M from Avery (1933).
(K) Transverse section through seed at the cotyledonary level (x70).
(L) Longitudinal-median section through seed just prior to endosperm rupture, showing ruptered testa (x50).
(M) Longitudinal-median section through seedling at 9 days (x50).
Avery GSJ (1933)
American Journal of Botany 20: 309-327
Structure and germination of tobacco seed and the developmental anatomy of the seedling plant.
Avery (1933) - Download a PDF file - 3.7 MB
|
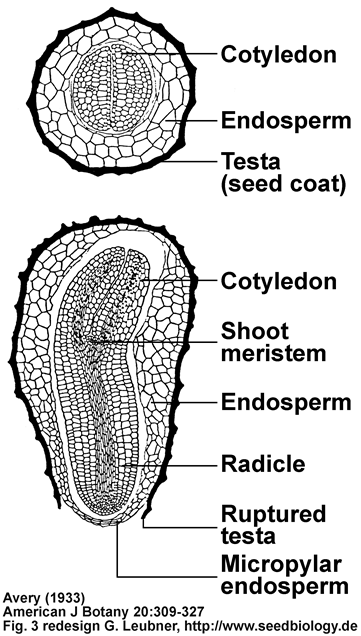 |
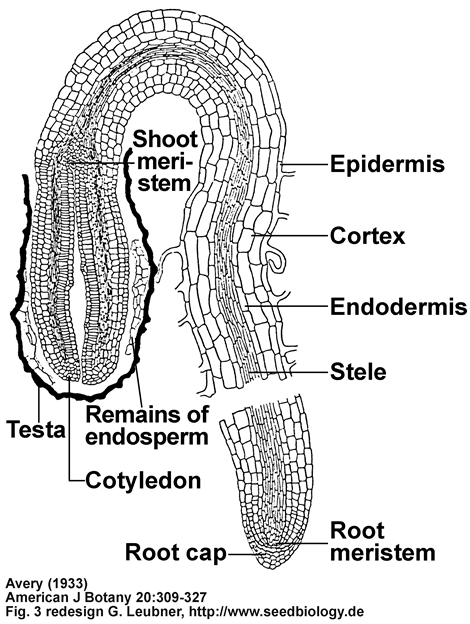 |
|
 |
|
|
Endospermic seed structure (Eudicots): Solanoidae subgroup of Solanaceae -
tomato and pepper as model systems in seed biology |
Tomato and pepper seeds are type members of the Solanoideae subgroup of Solanaceae.
In mature tomato (Lycopersicon esculentum) and pepper (Capsicum annuum) seeds the embryo is surrounded by an abundance of endosperm cells and by the testa (seed coat). The embryos are curved and flattened, the seeds are discoid, and a micropylar cap-like structure consisting of endosperm and testa covers the radicle tip. This micropylar cap of Solanoideae-type seeds is the place of radicle protrusion, but there is
no visible distinction beween testa rupture and endosperm rupture.
|
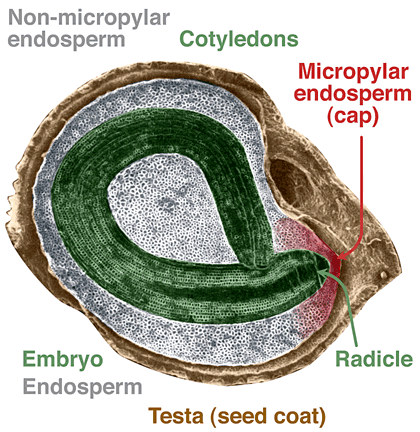 |
Drawing showing a mature seed of Capsicum annum (Color image by Katrin Hermann based on a EM image by Watkins and Cantliffe, Plant Physiol 72: 146-150, 1983). Color drawing published in Finch-Savage and Leubner-Metzger (2006). |
|
 |
Endospermic seed structure (Eudicots): Euphorbiaceae - castor bean - Ricinus communis |
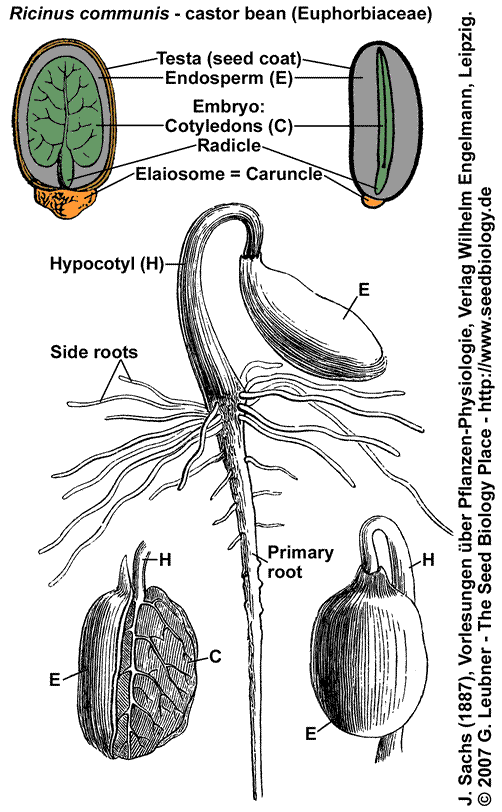 |
|
The castor bean (Ricinus communis) plant belongs to the Euphorbiaceae family (Rosid clade) and is a perennial shrub with large, palmately lobed leaves and sharply toothed leaf margins. The leaves are usually deep green, but in some strains they have a reddish cast. The fruit is a quarter-sized, round, spiny capsule, often reddish, containing up to three shiny, smooth, mottled seeds. FA1-type seed.
Castor bean seeds are highly toxic to humans and many animal, ingestion of two beans can be lethal to humans. The action of ricin, the toxic water-soluble protein, is well characterized. Ricin accumulates in the seeds, but is also found at lower concentrations in the leaves. The seed coat must be damaged to allow water to penetrate the seed interior for ricin to be absorbed in the intestines.
Castor bean seeds contain an elaiosome (caruncle, see figure). Elaiosomes are fleshy structures that are attached to seeds of many plant species. They are rich in lipids and proteins an have different shapes. In many cases elaiosomes attract ants, which disperse the seed (myrmecochory). The particular elaiosomes of Euphorbiaceae seeds are called caruncle. Like many other Euphorbiaceae seeds, Ricinus communis are carunculate; seeds without catuncle are called ecarunculate.
Castor bean seeds are endospermic (see figure), the embryo is spatulate axile, and the seed has physiological dormancy (PD). The cotyledons are thin and broad and the endosperm is the major storage tissue. Castor bean seeds are a classical system for studying endosperm reserve breakdown, especially for lipid and protein mobilization. The cells of the endosperm of castor bean seeds undergo programmed cell death after their oil and protein reserves have been mobilized. Castor bean seed germination is epigeal and the cotyledons of the seedling absorbe the nutrients from the endosperm, which encloses the cotyledons until it is obliterated. |
|
 |
|
|
Perispermic seed structure (Eudicots): Amaranthaceae -
sugar beet (Beta vulgaris) - a complex seed/fruit as disperal unit |
- Perispermic seeds and fruits: Perisperm is diploid maternal food storage tissue originates from the nucellus. Perisperm is found only in some species, e.g. in Beta vulgaris, Piper nigrum, Coffea arabica, many Caryophyllales. Sugar beet is emerging as a germination model for complex P-type seeds/fruits.
- Sugar beet fruits and seeds: In the achenes of monogerm cultivars of Beta vulgaris L. (Amaranthaceae, a family of the Caryophyllid clade of the core Eudicots) the ‘botanically true’ seed is surrounded by a thick pericarp (see below, Fig. 1, Hermann et al. 2007). The sugar beet pericarp is known as a fruit tissue that can restrict water and oxygen uptake by the enclosed seed. Except for the basal pore, the pericarp is composed of a dense, impervious layer of sclerenchyma cells. The operculum, i.e. the ovary cap of the fruit is the upper part of the pericarp; and the basal pore, i.e. a pore-like pericarp structure filled with loose cells at the bottom part of the pericarp have both been proposed as major entry points for water and oxygen. Removal of the operculum and/or the use of ‘isolated true seeds’ removed these restrictions and promoted radicle emergence. Based on the peripheral location of the embryo, the sugar beet seed can be structurally classified as being perispermic and P-type (Finch-Savage and Leubner-Metzger, 2006).
- Sugar beet seed proteome website: Hyperlink to the Seed Proteome Website (http://www.seed-proteome.com) of the
'Joint Laboratory CNRS / Bayer CropScience' - seed proteome lab of Dr. Dominique Job in Lyon (France) and the
'Laboratory of Seed Biology INRA / AgroParisTech' - Seed proteome lab of Dr. Philippe Grappin in Paris (France).

- Sugar beet germination - a comparative study of fruits and seeds (Hermann et al. 2007): Treatment with ethylene or the ethylene precursor 1-aminocyclopropane-1-carboxylic acid (ACC) promoted radicle emergence of sugar beet fruits and seeds. Abscisic acid (ABA) acted as an antagonist of ethylene and inhibited radicle emergence of seeds, but not appreciably of fruits. Sugar beet radicle emergence appears to be controlled by the pericarp, by ABA and ACC leaching, and by an ABA-ethylene antagonism that affects ACC biosynthesis and ACO gene expression. An embryo-mediated active ABA extrusion system is involved in keeping the endogenous seed ABA content low by 'active ABA leaching', while the pericarp restricts ACC leaching during imbibition.
|
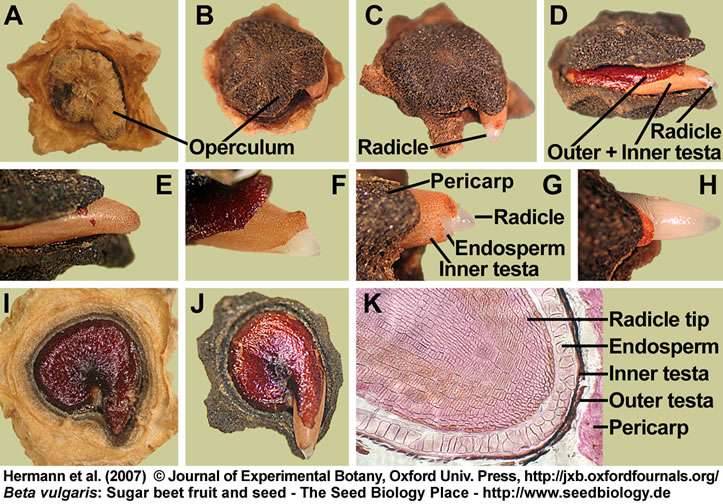
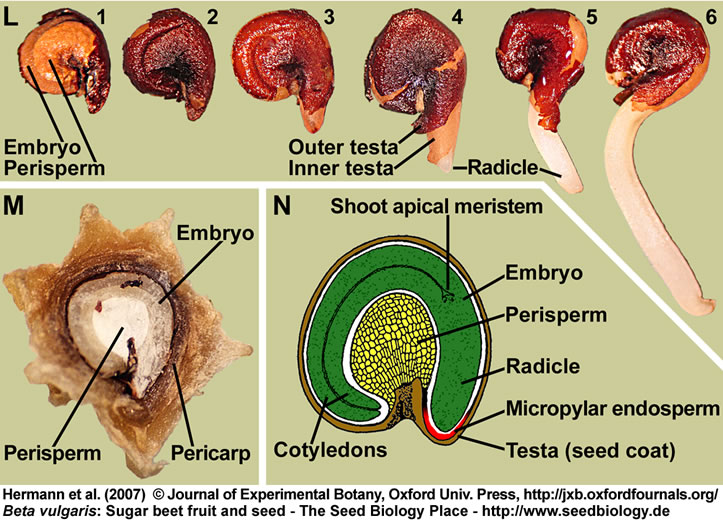 
|
Structure and germination of mature fruits and seeds of Beta vulgaris (sugar beet):
(A-H) Visible events during the incubation of sugar beet fruits in water:
(A) Dry fruit.
(B,E) Operculum opening; note that the radicle tip is still enclosed by the micropylar endosperm and the inner testa.
(C,D,F-H) Radicle emergence through the seed covering layers (testa and endosperm) is the completion of germination.
(I,J) Seed germination studied with deoperculated fruits. The sugar beet seed has a lentil-like structure (about 3 mm diameter and 1.5 mm thick) and occupies a horizontal position within the fruit.
(J) Radicle emergence through the seed covering layers (testa and endosperm) is the completion of germination.
(K) Microscopic section through a dry fruit showing the radicle tip enclosed by the covering layers.
(L) Distinct stages of sugar beet seed germination: Isolated dry seed (1,2); note that the testa was removed in (1) to make the embryo and perisperm visible. Imbibed seed showing rupture of the outer testa (3) and radicle protrusion through all the seed covering layers (4-6).
(M) Section through a mature sugar beet fruit. The curved embryo completely encloses the perisperm, which is dead starch storage tissue localized in the seed center.
(N) Drawing of a sugar beet seed; modified from Bennett and Esau (1936) and reproduced by the kind permission of the United States Department of Agriculture.
(A-N) Figure 1 from Hermann et al. (2007).
|
|
Endospermic seed structure (Monocots): Alliaceae - onion |
|
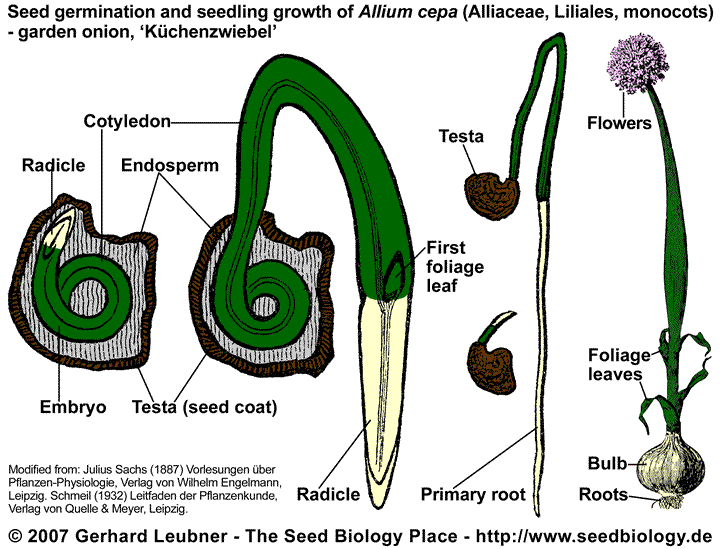 |

|
In monocot seeds like onion (Allium cepa, garden onion, bulb onion, 'Küchenzwiebel', Alliaceae, Liliales) the curved embryo has one cotyledon. It is embedded in endosperm tissue, which is the main food storage tissue of monocot seeds. The endosperm is surrounded by the testa (seed coat). Most Allium seeds have morphophysiological dormancy (MPD), they are therefore stored for several months to give their "underdeveloped" embryos time to grow (example: A. rothii). After (epigeous) germination the testa and endosperm of onions remains temporally attached to single cotyledon while the seedling primary root grows into the soil. The developing seedling obtains nutrients from the endosperm by way of the cotyledon. In addition, the green cotyledon of onion functions as a photosynthetic leaf, contributing significantly to the food dupply of the developning seedling. The plumule (young foliage leaves) emerges from the protective, sheathlike base of the cotyledon, elongates, and forms the foliage leaves of the seedling.
The wild progenitor of cultivated onion is not known, although it may be Allium vavilovii. The cultivated Allium cepa has been placed into two horticultural groups, the Common Onion group includes the typical bulb onion, and the Aggregatum group consists of those subspecies or varieties of A. cepa whose lateral buds have been active, thereby forming clusters of smaller bulbs, e.g. in shallots. Onion plants form thick bulbs consisting of scale leaves in response to specific day lengths and temperatures. The long-day onion plant requires a day length of at least fourteen hours to initiate bulb formation, and are grown in the northern latitudes including Europe, often from seed or transplants sown in early spring. Bulb formation takes place during the summer months, and bulb harvest is during the later summer and the fall. Other cultivated Allium species include garlic (A. sativum), leek (A. ampeloprasum), and Chinese chives (A. tuberosum). |
|
 |
Endospermic seed structure (Monocots): Poaceae (cereal grain, caryopsis) - wheat and other cereals |
|
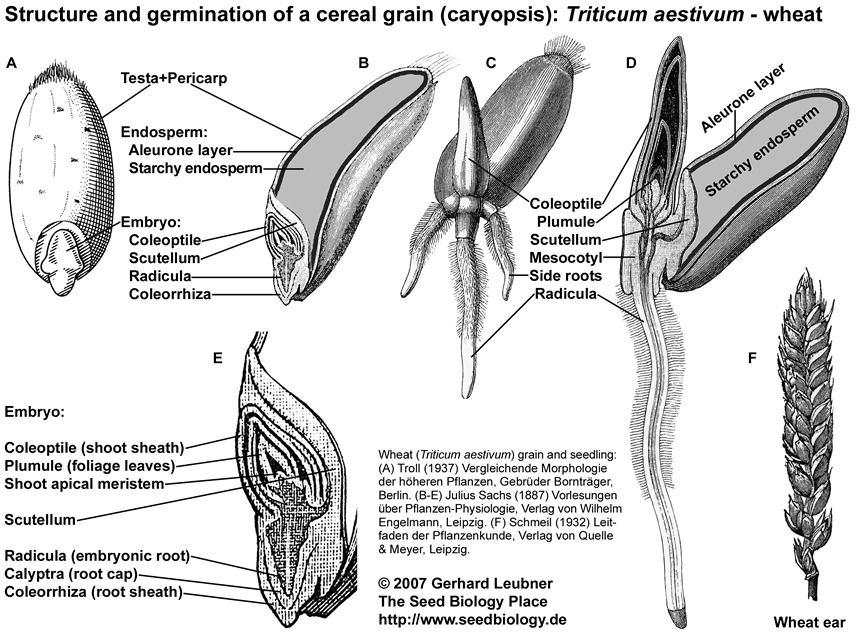
In cereal crops, a certain degree of physiological dormancy (PD) at harvest is a desirable trait because it prevents viviparous germination of grains in the head following exposure to cool moist conditions (Finch-Savage and Leubner-Metzger (2006). This pre-harvest sprouting (PHS) in many wheat cultivars, due to low harvest dormancy, has led to reduced grain quality at harvest and therefore serious economic losses. In contrast, high harvest dormancy of barley results in extra storage costs because the grain requires after-ripening to achieve the rapid and uniform germination required for the malting process. Thus, a defined level of seed dormancy is an essential component of seed quality. QTL analyses of cereal crop dormancy is one way to identify genes that underlie these physiological problems with PHS.
Red wheats are generally more dormant and sprout resistant than white wheats. Major genetic components used to decrease PHS in wheat are the red grain color (R) loci located on the 3A, 3B and 3D chromosomes. Dominant alleles promote expression of red pigments (phlobaphenes) within the testa/pericarp that tighly surrounds the embryo. It is not known whether the R genes provide increased dormancy or are very closely linked to other dormancy-increasing loci (Flintham 2000; Warner et al. 2000).
The role of the VP1 (viviparous1, ABI3 homolog) transcription factor in controlling PHS and ABA sensitivity has been investigated. VP1 controls high-pI alpha-amylase promoter and alternative splicing has been found by the group of Mike Holdsworth for cereal VP1 transcripts (McKibbin et al. 2002). In addition, PKABA1, a protein kinase, accumulates in mature wheat embryos and is responsive to ABA.
Seed dormancy of cereal grains is partially associated with the embryo (embryo dormancy) and partially with the covering layers (coat dormancy). Cereal coat dormancy is confered by the endosperm plus testa/pericarp and determines the timing of grain germinability (Benech-Arnold 2004). For example, sprouting-susceptible cultivars are those whose coat-imposed dormancy is terminated well before harvest maturity. Cereal seed dormancy is usually rather low in cultivated cereal grains. Wild oat, Avena fatua, and wild rice, Oryza sativa, are highly pernicious weeds and are cereal grain model with deep physiological dormancy (see references on the website of Mike Foley).
In some cereals (i.e. barley, rice), the glumellae (hull) adhering to the caryopsis represents another constraint for embryo germination in addition to that already imposed by endosperm plus tests/pericarp. Several mechanisms have been suggested as being responsible for dormancy in barley grain: ABA in the embryo and the ability of dormant embryos for de novo ABA biosynthesis upon imbibition has been proposed as a mechanism of barley grain dormancy. Release of dormancy is associated with the loss of this ability and a lower ABA sensitivity of the embryo.
It has also been suggested that hull-imposed coat dormancy is mediated by high polyphenol-oxidase activity existing in the barley glumellae which results in oxygen deprivation for the embryo. The limitation of oxygen supply to the embryo by oxygen fixation as a result of the oxidation of phenolic compounds in the glumellae (Benech-Arnold et al. 2006). This publication suggests that hypoxia, either imposed artificially or by the glumellae, increases embryo sensitivity to ABA and interferes with ABA metabolism, it mediates the inability of the embryo to inactivate ABA. An important role for ABA 8'-hdroxylase (CYP707A) in controlling barley dormancy and that the action of the enzyme is confined to the coleorhiza of cereals was proposed (Millar et al. 2006). Cyp707A genes encode ABA degrading enzymes (Kushiro et al. 2004) and affect Arabidopsis seed germination and dormancy (Müller et al. 2006).
|

|
Monocot species like wheat (see figure) and other cereal species have caryopses (cereal grains) as propagation units. Caryopses are single-seeded fruits in which the testa (seed coat) is fused with the thin pericarp (fruit coat). Cereal grains have highly developed embryos and in cereal grains the triploid endosperm consists of the starchy endosperm (dead storage tissue) and the aleurone layer (living cells).
Organs of the cereal embryo are the coleoptile (shoot sheath), the scutellum, the radicula and the coleorrhiza (root sheath). The scutellum is homologous to the leaf blade of the cotyledon and the coleoptile to the leaf sheath of the cotyledon. After germination, the scutellum functions in absorbing the nutrients from the starchy endosperm and delivering them to the growing seedling. After germination, the coleoptile protects the shoot meristem and plumule when growing through the soil. The coleorrhiza has a similar function for the radicle prior to germination. The coleorrhiza is the first structure that grows through the pericarp, it is then ruptured by the radicle (the completion of germination).
Germination and seedling establishment of cereal grains is hypogeous. After the primary root emerges, the coleoptile is pushed upward by elongation of the mesocotyl. The coleoptile elongates and reaches the soil surface. Here it ceases to elongate and the first leaves of the plumule emerge through an opening at its tip.
Mobilization of the food storage in the starchy endosperm is a post-germination event and requires an embryo signal (gibberellin) which induces the production and secretion of hydrolytic enzymes from the aleurone layer.
|
|
 |
|
|
|
 |
| |
|
|
|


 |